How does barley resist a wheat pathogen?
Scientists discover that the same gene can confer resistance to completely different pathogens
The fungal pathogen stripe rust Puccinia striiformis causes major global losses in cereal crop yields, particularly wheat. The species has independent lineages that infect diverse cereal species, such as wheat stripe rust (P. striiformis f. sp. tritici) and barley stripe rust (P. striiformis f. sp. hordei) which infect wheat and barley, respectively.
In a study published in Nature Communications, the authors set out to understand how barley resists infection by wheat stripe rust. The authors found that three resistance genes Rps6, Rps7 and Rps8 contributed to the immune response in barley towards the non-adapted wheat pathogen. More interestingly, they found Rps7 to cosegregate with barley powdery mildew resistance at the Mla locus, meaning they are inherited together. This shows that two distinct haplotypes of Mla have coupled resistance to the adapted pathogen barley powdery mildew and the non-adapted pathogen wheat stripe rust.
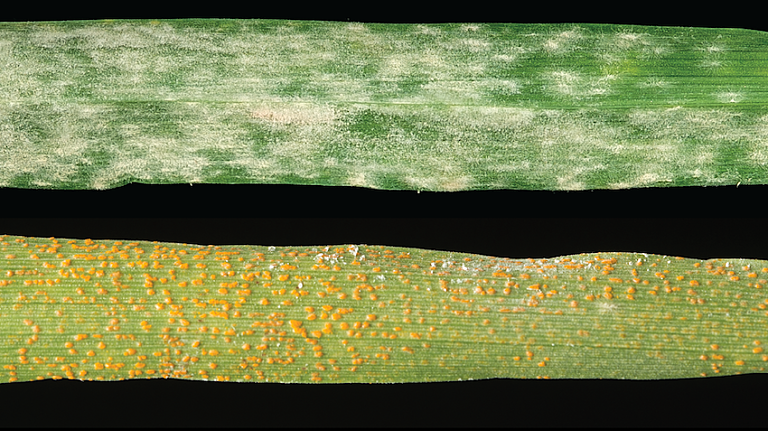
Barley powdery mildew and wheat stripe rust.
This raises the question: How can taxonomically diverse pathogens be recognized by the same resistance gene?
The plant immune system can be divided into passive and active barriers, the passive barrier being the physical barrier, such as the plant cuticle and cell wall. Within the cell, intracellular immune receptors provide an active barrier to pathogen invasion.
Nucleotide-binding leucine rich repeat (NLR) proteins such as Rps7/Mla are the largest class of intracellular immune receptors and have incredible sequence diversity. Some NLRs can directly recognise pathogens through proteins called effectors, which are secreted by the pathogen into the cell to supress the plant’s innate immunity or to assist in the acquisition of nutrients. Other NLRs indirectly recognize pathogens by guarding the host protein that is targeted by effectors. Through indirect recognition, an NLR could potentially recognize many different pathogens based on their interaction with the host protein.
After discovering that the same gene can confer resistance to completely different pathogens, the next set of questions will focus on how this works. One could therefore think of three different mechanisms underlying this multiple pathogen recognition:
(1) effectors from these pathogens have a conserved structure that allows them to directly interact with the host’s NLR
(2) the NLR can recognize different effectors by containing different binding sites, or
(3) the NLR indirectly recognizes pathogens by guarding a host protein that is a shared effector target by multiple pathogens.
Mounting evidence suggests that mildew Mla directly recognizes effectors, meaning that the third hypothesis is unlikely.
This research has scratched the surface of the complex and fascinating architecture underlying non-adapted resistance in cereals and provides an explanation for why plants can evolve immunity against non-adapted pathogens. The genetically coupled resistance for an adapted and non-adapted pathogen located at Mla means that the selection for an adapted pathogen, which provides a direct fitness benefit, could directly influence the plant’s resistance to other pathogens.
By not understanding coupled resistance, there is the risk of unintentionally introducing susceptibility genes or removing genes that protect plants against non-adapted pathogens. Therefore, these findings are particularly important when considering marker assisted selection and the fixation of resistance genes in plant breeding programs.
Jan Bettgenhaeuser, co-first author of the work stated that, “Host and nonhost resistance are often described as mechanistically separate. However, we were able to show that Mla provides resistance to both host and nonhost pathogens. This multiple-pathogen recognition is a fascinating piece in the puzzle of how plants are able to grow healthily, despite being exposed to an immense array of diverse pathogens on a constant basis.”
Matthew Moscou, who led the team stated that, “For me, one of the most exciting take homes from this work is understanding how an immune receptor can recognise completely different proteins. The evolution of plant and pathogen has created a rich tapestry that we are only beginning to understand. In the long-term, we hope to use this knowledge to discover new immune receptors and design principles for recognition.”
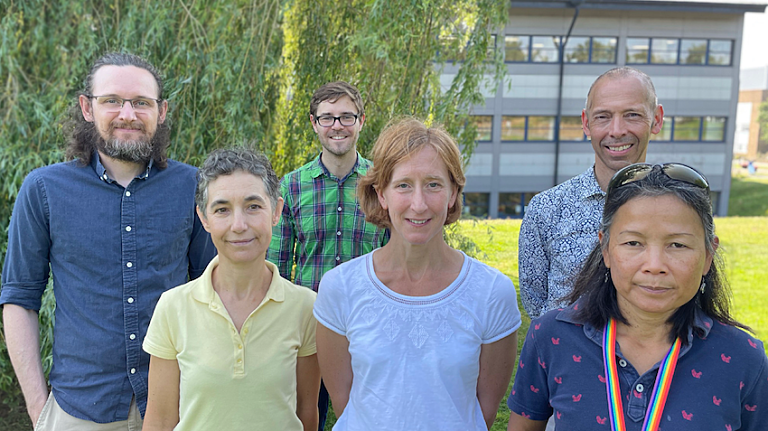
Matthew Moscou and his team, together with Matthew Smoker and Jodie Pike of the Tissue Culture &
Transformation team at The Sainsbury Laboratory.
The article “The barley immune receptor Mla recognizes multiple pathogens and contributes to host range dynamics” can be found here.
For more information, or to interview the paper’s authors, please contact Mia Cerfonteyn at comms@tsl.ac.uk
About The Sainsbury Laboratory
The Sainsbury Laboratory (tsl.ac.uk) is an independent research institute that focuses on plant health for a sustainable future. It makes fundamental scientific discoveries in molecular plant-microbe interactions and applies these to reduce crop losses caused by plant diseases, particularly in low-income countries. Around one hundred and twenty staff and students work and study at the Laboratory which is located on the Norwich Research Park, United Kingdom. The Laboratory is generously supported by the Gatsby Charitable Foundation and by the University of East Anglia, wins competitive grants from the BBSRC, ERC and other research grant funding bodies and, for some research programmes, is funded by commercial companies. Established in 1987, highlights of The Sainsbury Laboratory include: discovery of RNA interference in plants by Prof. Sir David Baulcombe FRS as recognised by the Lasker Award and the Wolf Prize in Agriculture, discovery of the first immune receptor in plants by Prof. Jonathan Jones FRS, three current Group Leaders are Fellows of the Royal Society, and five researchers who have been on the Highly Cited Researchers list of top 1% scientists in the world since 2018.